Published in 1/2024 - The Heart of the City
Urban Greenery as a Climate Action
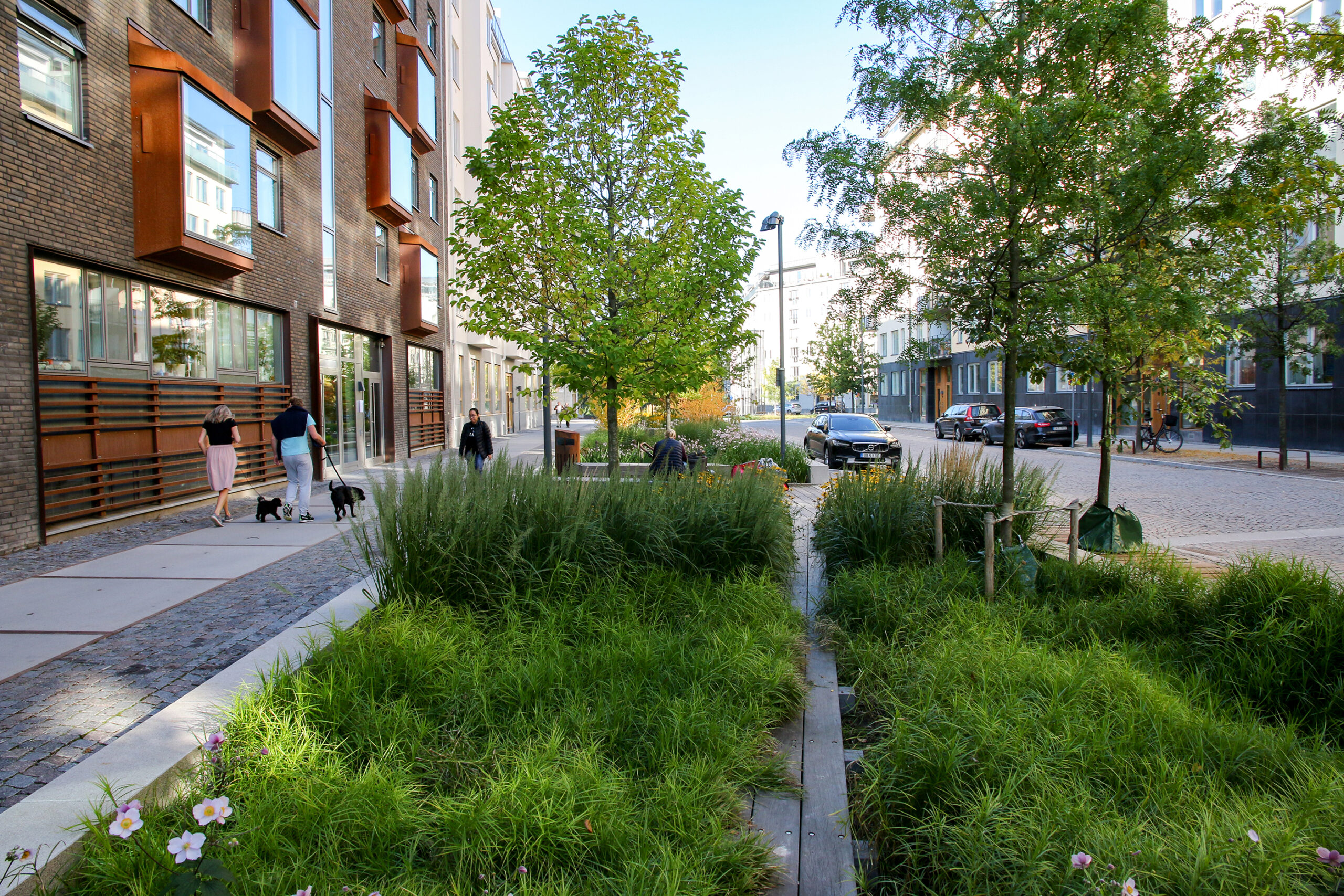
To date, urban planning has failed to pay due consideration to urban carbon sinks and the numerous other benefits of urban green. Researchers in the Co-Carbon project compiled a set of tools for architects and landscape architects to foster carbon-smart solutions.
The EU is committed to achieving carbon-neutrality by 2050, and Finland has set an even more ambitious target of 2035. According to the carbon-neutrality objective, the permitted carbon dioxide emissions may not exceed the amount that can be bound from the atmosphere into carbon sinks. While this does entail a significant reduction in emissions, it also requires the establishment of more efficient carbon sinks. The sinks have received plenty of attention in the field of forestry, in particular, but they are also significant in an urban environment. Our ongoing Co-Carbon research project reveals that in Helsinki, for instance, slightly less than half of the city’s carbon sequestration and storage (CSS), or the binding of carbon in layman’s terms, is based on urban forests and the rest on other green infrastructure.1 This is an interesting finding, as it highlights the fact that not only forests but also parks, meadows, gardens and street plantings bind carbon. At the same time, this result opens up new possibilities for architects and landscape architects to figure out how to design carbon-smart urban green infrastructure, thereby making both carbon sinks and carbon stores more efficient.
The CSS potential of urban vegetation and soil has been significantly less studied than the carbon cycle of forests or agricultural areas. The carbon process of urban nature is far more complicated than that of a natural forest, as urban green consists of small-scale, mosaic elements. The core science is, however, the same. Vegetation binds carbon dioxide from the atmosphere by growing leaves, stems, trunks and roots. Through the degeneration of biomass and cell respiration, part of the carbon dioxide is then released back into the atmosphere, while the rest is stored in plant parts and the soil. A carbon sink forms if the amount of carbon stored exceeds that of the carbon that is released.
Unlike in forests, the carbon cycle and storage in the built environment is influenced by several factors related to the growth environment and to human activity. For example, the space reserved for roots and canopy affects a tree’s possibilities of serving as a carbon sink. A good rule of thumb is that the better a tree is able to thrive, the better it is also able to photosynthesize and bind carbon from the atmosphere. With CSS of urban greenery, we must also consider the effect of emissions from construction and maintenance – for example, the establishing of growing media and the cutting of lawns.2
Not only forests but also parks, meadows, gardens and street plantings bind carbon.
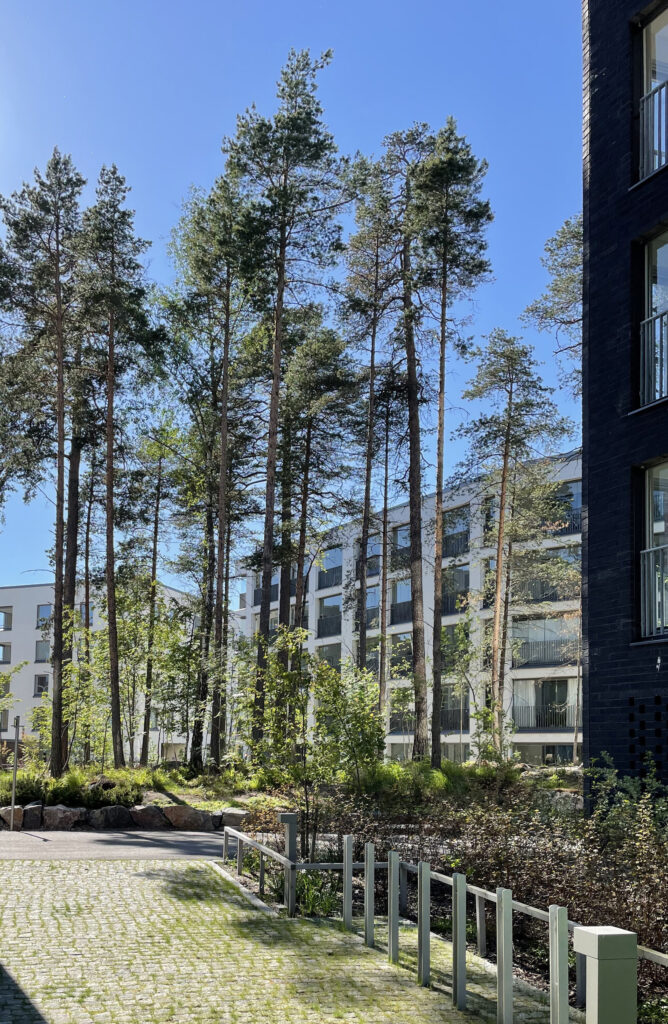
Generally speaking, the carbon discussion tends to be dominated by specific perspectives, while others remain overlooked. Most typically, this can be seen as an emphasis on emissions and a disregard for carbon sinks in the urban environment. Urban green may seem like a minute factor to be considered in the vast overall context of climate work. Another blind spot is the significance of soil as a carbon store. Based on the carbon sink survey conducted by the Helsinki Region Environmental Services for example, the carbon storage capacity of the area’s soil is roughly double that of the area’s vegetation.
A third perspective deserving of more attention is the CSS capacity of various types of vegetation, such as shrubs, meadows and lawns. Trees are generally recognized as carbon sinks, but other types of vegetation are also relevant. A square metre of lawn, for example, has been shown to bind nearly half the amount of CO2 from the atmosphere that a square metre of Finnish forests is able to bind.3 However, this finding does not cover the emissions caused by lawn maintenance, such as mowing or fertilizing.
A fourth perspective that often remains overlooked in the climate discussion is the multifunctionality of urban greenery, even though this is highly significant, particularly from the point of view of climate adaptation. In addition to CSS, urban green mitigates heat waves and floods in the city, supports human wellbeing and also offers habitats for several other species. Urban green, therefore, offers solutions for both the climate crisis and the loss of biodiversity.
These little-discussed perspectives and opportunities for fostering carbon-smart solutions can be summarized in the form of three cornerstones: preserving carbon storages, increasing carbon sinks, and promoting low-emission landscape construction and maintenance.
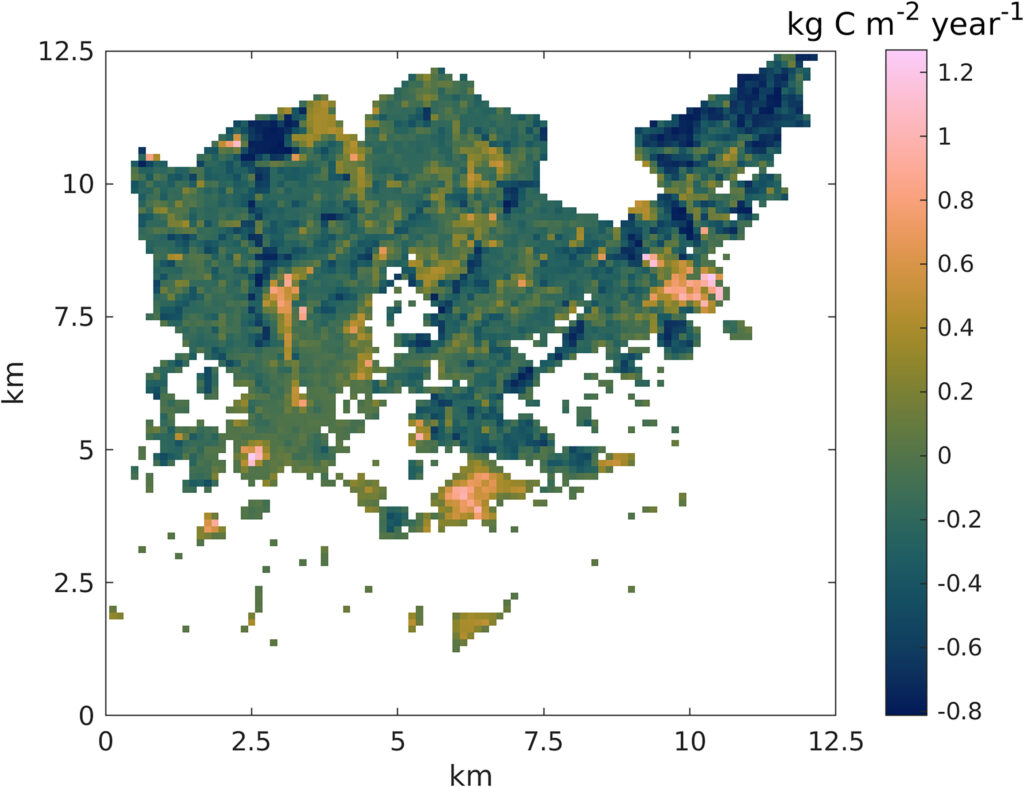
1. Preserving carbon storages
The climate benefits yielded by urban green are directly dependent on the area covered by vegetation, as well as its ability to store carbon. Existing vegetation and soil constitute the most important carbon store in an urban environment. This is why their preservation is the primary means in urban planning to promote carbon-smart solutions.4 Areas with a significant carbon storage capacity, forests in particular, are often also important in terms of biodiversity. An illustrative example is Helsinki’s “green fingers”, the radial green zones which, according to research results, support both CSS and biodiversity.5 The enhancing of synergies is therefore important.
The life cycle of urban green is an essential element of carbon storage in the long term. The longer that trees are allowed to grow, the larger their carbon store will also grow to be. This also compensates for the peak in emissions occurring during the early phases of planting, which is caused by the release of carbon through the degeneration of organic matter in the growing media. Over time, carbon is accumulated back into the soil and plant parts, but it may take years before the planted vegetation begins to serve as a carbon sink. This is why we must especially seek to preserve mature trees within urban environments.
Carbon sinks are typically considered at the level of an entire city or a region, but the other climate benefits of urban green infrastructure are more local. Mitigation of the urban heat island phenomenon and of urban floods, for example, is required at the neighbourhood level, as is also the case with recreational benefits. The quantitative objectives of urban green and related climate benefits are directly linked with the density within the urban fabric. A good example of this is Helsinki, where the tree canopy cover, i.e. the area of arborescent vegetation of more than two metres in height, varies by city district from some 4 to roughly 65 percent.6 As a point of reference, one might mention that the international recommendation for the canopy cover of city districts is 30%.7 Although it is not possible or even reasonable to make all city districts equally green, it is useful to shine a light on the local benefits of green infrastructure and to also learn from previous urban planning practices. The sparsely built and lush forest suburbs of post-war Helsinki, for example, stand out in regard to the mitigation of urban heat islands and CSS capacity, when compared to high-rise city districts from other periods.8 This shows that the climate impacts of urban planning should not be evaluated solely from the point of view of construction and reducing emissions.
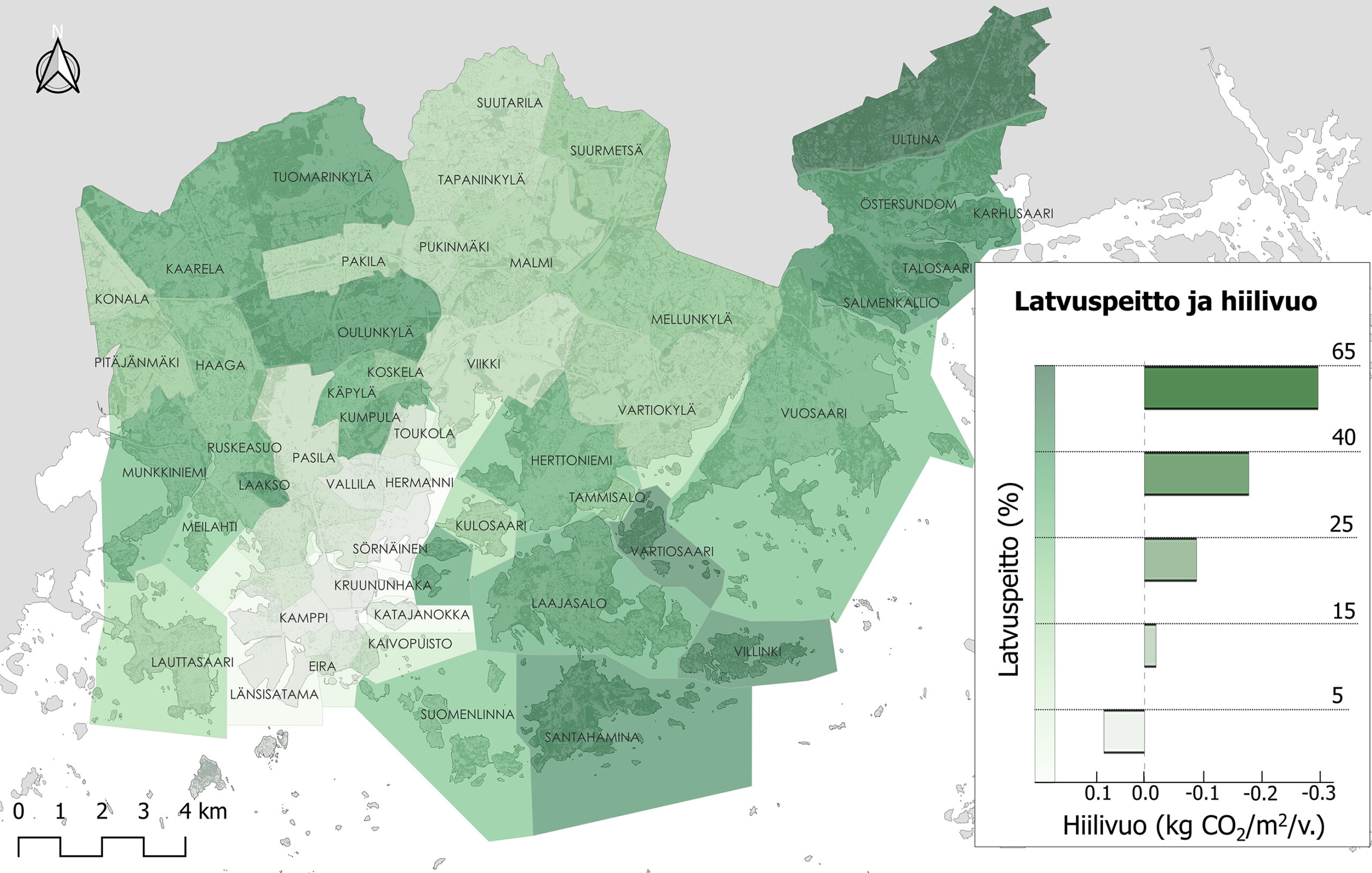
The urban green in Helsinki is not distributed evenly. The canopy coverage (area covered by arborescent vegetation of more than 2 metres in height) varies by city district from 3.6 to 63 percent. A darker tone indicates more canopy coverage. The areas begin to serve as distinct carbon sinks when the canopy coverage exceeds 15%–25%. Image: Antti Kinnunen (Source: HSY land cover data, University of Helsinki carbon flux data)
2. Building new carbon sinks
Alongside the preservation of existing green infrastructure, carbon sinks must be increased wherever possible.The climate benefits of green areas are particularly necessary in a dense urban fabric, and many large cities have woken up to this reality. In Paris, for instance, the objective of the ambitious Plan Arbre is to plant 170,000 trees within the city over a period of six years. The impact of the number of trees and of carbon-smart construction solutions on carbon sinks is undisputed. For example, in our study on block yards in Helsinki’s Kuninkaantammi residential development, we demonstrated that increasing the number of trees to be planted and utilizing biochar in the growing media tripled the capacity of the carbon sink formed by the residential yards, when compared to the implemented yard plans.9 Furthermore, in the modelling of carbon sinks in Tampere’s Hiedanranta district, we also discovered that various landscape construction solutions can at least triple the carbon sinks when compared to the preliminary plans drawn up for the area. The density and species of large trees to be planted had the greatest impact.10
When building new urban areas, it is important to guarantee favourable long-term growth conditions for urban greenery. This entails sufficient space for the canopy and roots, as well as suitable humidity and sunlight conditions. Special attention must be paid in planning to optimizing the area covered by vegetation and to generating multi-species and multi-layered vegetation areas.[11] A new type of controlled wildness in urban nature yield benefits in terms of both the climate and biodiversity. This kind of approach can be found in microforests, which are based on a method of reforestation originally developed by Japanese botanist Akira Miyawaki and have led to numerous applications in different parts of the world. Microforests are small, densely planted urban groves that can enhance the synergies of carbon binding and biodiversity.12
The choice of building technique and maintenance method for urban green infrastructure influences the carbon storage capacity. During the construction phase, the priority is to preserve the soil and its carbon storages. This also supports the beneficial microbes found in the soil which foster the growth of vegetation and thereby also the binding of carbon. Such microbes are typically lacking in engineered growing media. The carbon storage of the soil can also be influenced through maintenance practices – for example, it is not always wise to remove leaf and mowing litter, as it feeds the carbon stored within the soil.
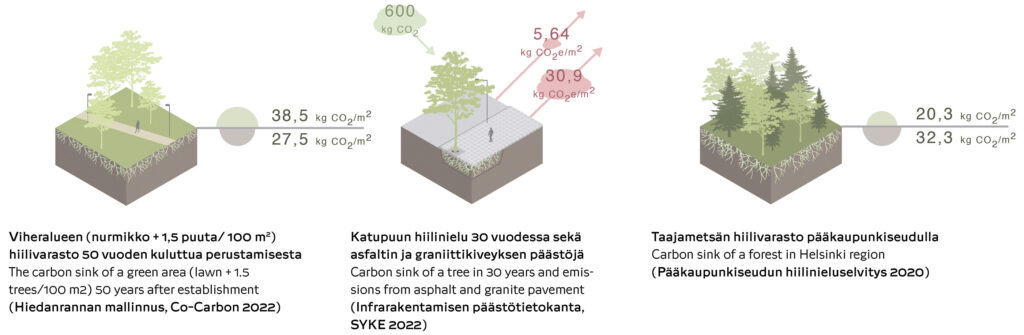
The role of soil is significant, when assessing the carbon storages of different vegetation types. Due to a lack of established calculation methods, however, there are no unambiguous results as regards vegetation and soil. Photo: Mari Ariluoma, Lotta Lipsanen
3. Low-emission construction and maintenance methods
The promotion of low-emission practices is rapidly becoming a standard in the construction sector, but little is known to date about such practices and principles when it comes to vegetation and the soil. As an example, the national emission database for construction, which is used to calculate the carbon footprint of construction, currently includes a very limited reference base for landscape construction products. The database is being developed as regards nursery products and growing media data. Indeed, the low-emission objective should apply to the materials and methods of environmental construction and maintenance in the same way that it does to other building products. The utilization of recycled soil as well as biochar and various solutions based on a circular economy, for instance, can mitigate the substantial emissions caused by growing media and increase the amount of carbon stored. It is also advisable to evaluate the maintenance of green areas from the perspective of emissions and to revise current practices to make the fostering of carbon sinks the objective of care and maintenance.
The promotion of low-emission practices requires the development of life cycle assessment methods for the landscape industry, particularly when it comes to vegetation and soil. Life cycle assessments enable us to weigh where and how to build – heavy earthworks, growing media and various paving materials, for example, increase the emissions from landscape construction.13 An understanding of the life cycle and carbon sink impacts of urban green is also helpful when comparing various materials and implementation methods. This allows us to illuminate the climate benefits of planted versus paved areas or ground-level versus raised deck yards.
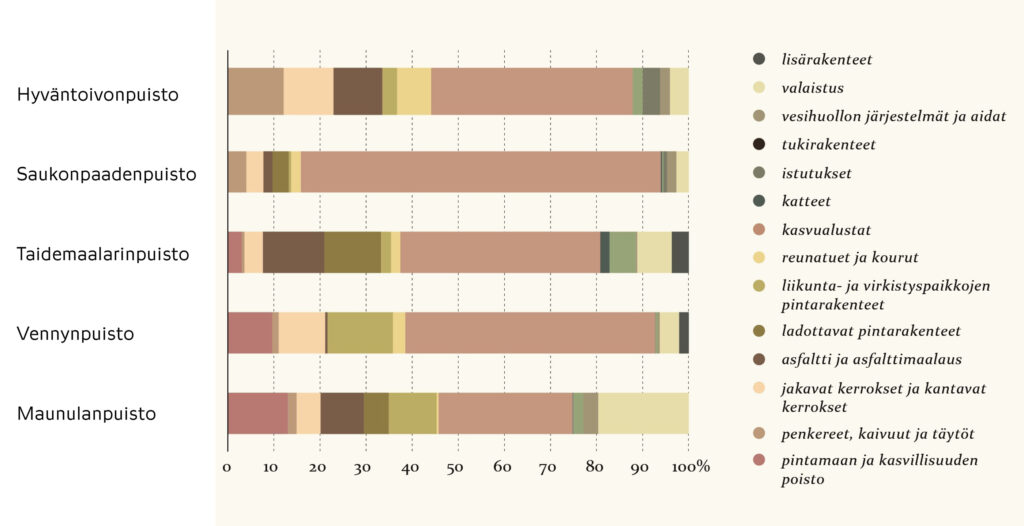
The emissions of the construction of five parks in Helsinki are calculated based on various construction elements and their percentage shares. Despite the differences between the parks, a common feature is growing media (light brown) as a significant source of emissions. The calculation does not include the transportation of vegetation, maintenance, or the construction of the park, except for the removal of vegetation and soil. Diagram: Caroline Moinel
More Effective Guidance for Carbon-Smart Practices
Carbon-smart urban green infrastructure can be promoted on several levels of planning. All climate work relies on legislation, but when it comes to green infrastructure, the current legislation has several gaps. The interpretation of the current Land Use and Building Act as regards green areas is narrow and mainly focusses on recreation. It would be necessary to incorporate the significance of green infrastructure as a multi-benefit climate solution into the new land use act that is currently being reformed. There is also work to be done on the climate change act. The section steering the climate action plans of municipalities and the related guidelines largely focus on emissions, while carbon sinks have been left out of the minimum requirements, which is bound to have a direct impact on the emphases in future municipal climate work. It would be necessary to set quantitative targets for carbon sinks and stores in the same manner as has been done for reducing emissions.
More comprehensive tools are also needed for evaluating climate impacts in land use planning. The impacts of urban planning on carbon sinks are rarely assessed, largely due to a lack of calculation models and research-based data. However, methods are currently being developed for this purpose, with the AVA method for assessing climate impacts of local detailed plans as an example. The carbon sequestration and storage capacity of green infrastructure can also be considered in the design of individual plots. One good tool is, for instance, the Green Factor method, for which our research project is developing a carbon calculator. It is also possible to account for CSS and low-carbon environmental construction in environmental certifications and procurement criteria, starting from the planning and extending all the way to the material purchases.
When it comes to green infrastructure, the current legislation has several gaps.
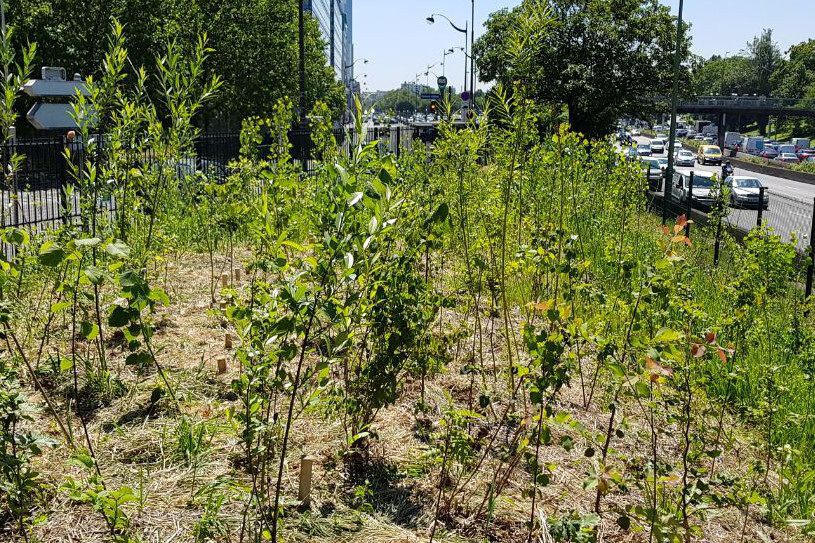
The importance of urban green has increased over the last few decades, and it is also increasingly considered to have strategic significance. Despite this, when it comes to actually investing in urban green, additional justifications and evidence of immediate cost benefits continue to be required. As it stands, the carbon sinks of urban green and their co-benefits are not yet sufficiently considered in urban planning. We are beginning to have the necessary knowledge and the general will to take action but the attitudes, processes, practices and methods are still wanting. What will eventually drive change is acknowledging urban green as critical infrastructure and as a necessary means to combat and prepare for climate change, to prevent the loss of biodiversity and to enhance the wellbeing of people as well as other living species and our planet. When we take care of the urban green, it will, in turn, take care of us. ↙
RANJA HAUTAMÄKI is an Associate Professor of Landscape Architecture at Aalto University, and MARI ARILUOMA is a doctoral researcher in landscape architecture and a partner at the landscape architecture firm Nomaji.
The article is based on the Co-Carbon research project (2020–2026), which is funded by the Strategic Research Council of the Academy of Finland (grant 335201, 335202). Collaborating in the project are the University of Helsinki, Aalto University, the Finnish Meteorological Institute, the Häme University of Applied Sciences and the University of Copenhagen. The authors wish to thank Elina Alatalo for comments on the article.
1 Minttu Havu, Liisa Kulmala, Hei Shing Lee et al.: “CO2 uptake of urban green in a warming Nordic city”. Manuscript in review.
2 Mari Ariluoma, Paula-Kaisa Leppänen, Outi Tahvonen et al.: “A Framework for a Carbon-Based Urban Vegetation Typology – A Thematic Review.” Environmental development 47, 2023.
3 Based on the studies conducted at Ossinlampi by Leena Järvi et al. in the Co-Carbon project.
4 Ranja Hautamäki, Leena Järvi, Mari Ariluoma et al.: Carbon-smart Urban Green Infrastructure as a Climate Solution: Policy recommendation for municipalities. 2023.
5 Christopher Raymond, Alex Lechner, Minttu Havu et al.: “Identifying Where Nature-Based Solutions Can Offer Win-Wins for Carbon Mitigation and Biodiversity across Knowledge Systems.” npj Urban Sustainability 3(1) 2023, 27–13.
6 Based on the calculations by Antti Kinnunen in the Co-Carbon project.
7 Nordic Council of Ministers: Nordic Cities. Green, Resilient, Healthy. Fostering national policies and initiatives for urban green space. Policy brief 2022.
8 Paula-Kaisa Leppänen, Antti Kinnunen, Ranja Hautamäki et al.: ”Impact of changing urban typologies on residential vegetation and its climate-effects – a case study from Helsinki, Finland”. Manuscript in review.
9 Mari Ariluoma, Juudit Ottelin, Ranja Hautamäki et al.: “Carbon Sequestration and Storage Potential of Urban Green in Residential Yards: A Case Study from Helsinki”, Urban forestry & urban greening 57, 2021.
10 Leena Järvi, Liisa Kulmala, Minttu Havu et al.: Hiedanrannan hiilinielut ja hiilinielujen lisäämisen keinot. Raportti Report 2023.
11 Ranja Hautamäki, Leena Järvi, Mari Ariluoma et al. 2023.
12 Anna Pursiainen: Näe metsä puilta – mikrometsästä Keravan hiilimetsäseen. Master’s thesis in landscape architecture, Aalto University 2023.
13 Caroline Moinel, Matti Kuittinen & Ranja Hautamäki: ”Estimating carbon flows in urban parks with life cycle approach. A case study in Helsinki”. Manuscript in review.